Research

Harnessing Disorder to Shape the Future of Technology.
The growing demand for advanced technologies across a wide range of applications drives the need for novel materials that can set new standards of performance. Our research focuses on the synthesis and characterization of these cutting-edge materials, emphasizing the fundamental understanding of structure-process-property relationships resulting from engineered disorder in advanced oxide ceramics. By leveraging diverse synthesis methodologies combined with a suite of advanced, multi-lengthscale characterization, we aim to unlock the full potential of these complex systems. Below, we highlight some of our current research initiatives!
NSF-DMR Award No. 2011839
High-entropy crystals, engineered through chemical complexity, exhibit unique properties and behaviors that are not found in more ordered materials. These materials follow unconventional crystal chemistry rules, leading to promising new functionalities. Our research group (IRG2) aims to identify and understand these rules by integrating theory, synthesis, characterization, and computation. We hypothesize that high configurational entropy allows atoms to occupy "misfit" environments, creating a range of local energies and disordered structures that lead to new macroscopic properties. Our goal is to discover how local atomic arrangements affect overall material behavior and to establish predictive guidelines for designing high-entropy crystals.
We explore the limits of incorporating misfit cations into a high-symmetry structure to observe how these affect material properties. Our overarching modeling effort integrates machine learning to predict structure, defects, and properties at multiple scales. At smaller scales, first-principles calculations predict local distortions and link them to properties like ion migration barriers, defect energies, and polarizability. This data then supports larger-scale phase-field and stochastic models to connect formulation, structure, and cooperative responses, providing insights into material design and functionality.
This IRG is in collaboration with The Pennsylvania State University and University of Michigan.

NSF-CMMI Award No. 2346484
Developing new methods to manufacture electronic materials with useful properties that do not occur naturally is a key toward technological advancement. The focus is on hafnium oxide, a material that can be engineered to exhibit spontaneous electric charge separation, making it valuable for next-generation low-power computing and memory devices. However, achieving this particular atomic arrangement is challenging, as hafnium oxide often contains impurities and more stable crystal phases that hinder its performance.
This project aims to overcome these challenges by developing a scalable method to create phase-pure ferroelectric hafnium oxide and hafnium zirconium oxide using atomic layer deposition (ALD). By employing plasma-enhanced ALD, researchers will create amorphous films and use techniques like X-ray diffraction and spectroscopy, electron microscopy, and electrical measurements to understand how processing conditions affect atomic structure and subsequent crystallization. The ultimate goal is to establish a precise process for creating high-purity materials, which could significantly advance the development of efficient, low-power computing elements, contributing to energy savings, economic growth, and enhanced national security.
This research is a collaborative effort with the University of Virginia, University College Dublin, and Queen's University Belfast.

ARL Cooperative Agreement No. W911NF-23-2-0228
Integration of acoustic, magnetic, and infrared sensing functions is achievable on PMN-PT piezoelectric crystals and films, using either heterogeneous integration or common substrate deposition and microfabrication. Research will explore heterostructure layer composition, deposition methods, epitaxial constraints, and electrode designs to optimize signal transduction, noise reduction, and bandwidth for each sensor mode. Integrated multi-sensing capabilities will allow noise rejection through gradiometric subtraction, enhancing detection in contested environments.
These sensors will share a common volume, enabling efficient threat detection, as multiple sensor types must be spoofed simultaneously for undetected intrusion. The integration will improve signal-to-noise ratio (S/N), lower power consumption, and provide reliable detection with sensor fusion, supported by new detection circuitry.
This research is in collaboration with The Pennsylvania State University and the Army Research Laboratory.

Thank you to all of our collaborators, past and present...

...and for all of the support through start-up, state, and federal funding.
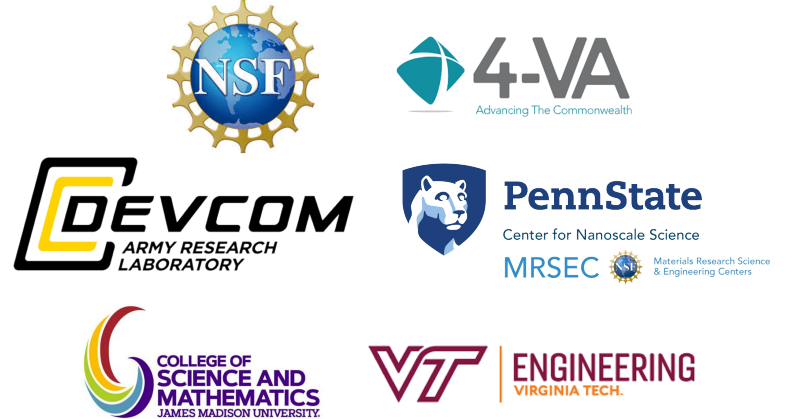